Neutron Structure Unveiled: Advancements in Physics
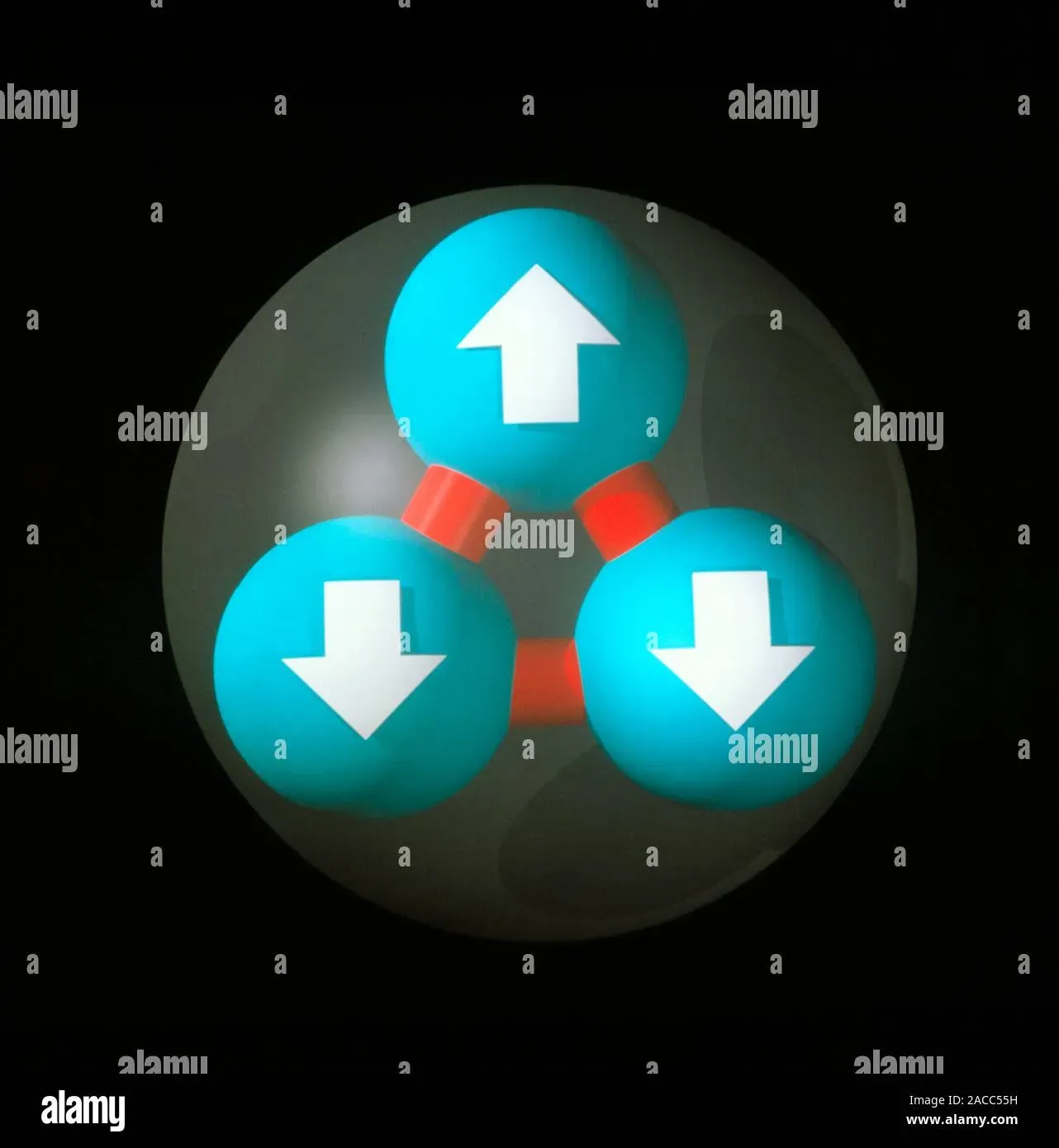
Neutron structure is a crucial element in the study of fundamental particles within atomic physics. Recent advancements have allowed physicists to delve deep into the intricacies of neutron particles, revealing their composition and the role they play as nucleons in the atomic nucleus. Groundbreaking research has employed new detection methods to measure deeply virtual Compton scattering on the neutron for the first time, providing unprecedented insights into particle physics. Understanding the neutron’s structure significantly enhances our comprehension of quantum chromodynamics (QCD) and the interactions between quarks and gluons. This pivotal work not only broadens our knowledge of neutrons but also opens up new horizons in neutron research, potentially impacting fields such as nuclear physics and astrophysics.
The analysis of neutron characteristics offers a fascinating glimpse into the building blocks of matter. By studying these electrically neutral particles, scientists can unravel the complex nature of nucleons, which include both neutrons and protons. This innovative exploration of deeply virtual Compton scattering sheds light on the interactions central to particle physics and reinforces the principles of quantum chromodynamics. As researchers continue to investigate the internal makeup of neutrons, the implications of their findings may extend beyond traditional physics, influencing areas such as cosmology and the development of new technologies. Ultimately, deepening our understanding of the structure of neutrons paves the way for future breakthroughs in diverse scientific domains.
Understanding Neutron Structure through Compton Scattering
Neutron structure has long been a pivotal area of study in particle physics, particularly due to the neutron’s role as a fundamental building block of atomic nuclei. This recent research utilizing deeply virtual Compton scattering has unveiled the intricate details of the neutron’s internal composition, something that has eluded physicists for decades. By analyzing how photons interact with neutrons, scientists can now start to map out the distribution of quarks and gluons inside these neutral particles, propelling our understanding of nucleons to unprecedented levels.
The significance of this study extends beyond just the discovery of neutron structure; it provides experimental validation of theoretical models rooted in quantum chromodynamics (QCD). As our understanding of neutrons deepens, we gain critical insights into how these particles interact with protons and how they contribute to the overall stability of atomic matter. This knowledge could alter existing paradigms in nuclear physics and influence how we view the interactions that underpin the universe.
Frequently Asked Questions
How does neutron structure relate to Compton scattering in particle physics?
Neutron structure is crucial in understanding particle physics, and Compton scattering is a technique used to probe it. By measuring deeply virtual Compton scattering on the neutron, physicists can gain insights into its internal structure, revealing how nucleons interact and combine to form the atomic structure of matter.
What role does quantum chromodynamics play in understanding neutron structure?
Quantum chromodynamics (QCD) is essential for explaining the strong interactions between quarks and gluons, which make up neutrons. Understanding neutron structure through QCD helps physicists elucidate the forces that bind nucleons together and impacts our knowledge of matter under extreme conditions, such as in neutron stars.
What are the implications of neutron structure research for modern physics?
Research into neutron structure has profound implications for modern physics, particularly in fields such as nuclear physics and astrophysics. The insights gained could reshape existing theories and models of atomic interactions and lead to advancements in various applications ranging from quantum computing to materials science.
Why is understanding the neutron structure important for particle physics?
Understanding neutron structure is vital in particle physics because it provides direct insights into the behavior of nucleons within atomic nuclei. This knowledge enhances our comprehension of the fundamental forces and particles that constitute matter, ultimately contributing to a deeper understanding of the universe.
How does neutron research influence advancements in quantum computing?
Neutron research influences quantum computing by exploring the fundamental properties of matter at a subatomic level. Insights gained from studying neutron structure can lead to the development of new materials and techniques that enhance the performance and stability of quantum computing systems.
Key Points |
---|
Physicists measured deeply virtual Compton scattering on the neutron for the first time, revealing nucleon structure. |
The study provides direct insights into neutrons’ inner workings, impacting particle physics and matter understanding. |
Published on April 10, 2025, by Joshua Shavit, marking a significant milestone in physics research. |
Investigates quantum chromodynamics (QCD) to explain quarks and gluons interactions under extreme conditions. |
Findings could reshape models in nuclear physics, astrophysics, and cosmology. |
Research on neutron’s internal structure opens new avenues for fundamental physics exploration. |
Insights could lead to advancements in quantum computing and materials science applications. |
Summary
Neutron structure is a key area of research in particle physics that reveals the foundational components of matter. Recent advances in measuring deeply virtual Compton scattering on neutrons have provided unprecedented insights into their inner workings. This study not only enhances our understanding of neutrons, which are essential constituents of atomic nuclei, but also promotes further investigations into quantum chromodynamics (QCD). The implications of these findings stretch across various fields, from nuclear physics to cosmology, potentially revolutionizing how we comprehend matter at its core.